“FOR IMMEDIATE RELEASE
🔬REVOLUTIONARY BREAKTHROUGH SETS HYDROGEN ENERGY IN A WHOLE NEW LIGHT
[City, Date] – Today marks an unprecedented achievement in the sphere of renewable energy! We’re incredibly excited to announce a thrilling breakthrough in hydrogen electrolysis that could totally redefine the landscape of the global energy sector. Brace yourselves, friends of tech, because you’re about to be blown away by this game-changing, phenomenal advancement.
This isn’t just a step forward; it’s a colossal leap towards a future brimming with sustainable energy! Our team of wizard-like scientists and technical magicians have unlocked the secrets of hydrogen electrolysis, achieving unprecedented levels of energy efficiency that were previously considered the stuff of dreams.
The newly discovered process, affectionately dubbed “Hydrogen 2.0,” operates at breathtakingly high efficiency levels. We’re not just talking 20%, 50%, or even 80%… Nope! Our revolutionary Hydrogen 2.0 is astonishingly hitting near-perfect efficiency rates! Imagine the capabilities of an energy source that delivers virtually no energy waste – it’s mind-boggling!”
(The preceeding delicious #hopium emission was courtesy of ChatGPT as expertly wrangled by human intelligence Michael Barnard)
In the past few years there have been at least two prominent and at least somewhat credible claims that people have 95% (HHV) efficient electrolyzers working in the lab.
So that’s my argument against hydrogen on the basis of inefficiency shot to pieces, isn’t it? Guess it’s time to pack up the @hydrogen science coalition and jump on the hydrogen as a fuel bandwagon, eh?
Let’s be clear: the problem with hydrogen isn’t that electrolysis is inefficient, or fuelcells are inefficient, or storage is inefficient. The problems with hydrogen as a fuel or energy storage medium are a) exergy destruction b) too many steps, each with efficiency limits and c) the properties of the H2 molecule.
Exergy Inefficiency
Exergy has a more complex definition to a thermodynamicist, but to a layperson or an average engineer, it’s safe enough to just call it “the potential to do work”. While a joule of room temperature heat and a joule of electricity are both 1 joule of energy, the former has ZERO exergy and is basically worthless if you have more of it than you need, and the latter is nearly pure exergy. You can convert electricity with very high (nearly 100%) efficiency into thermodynamic work, i.e. mechanical energy. As Michael Liebreich says, riffing on Orwell’s Animal Farm: “All joules are created equal, but some joules are more equal than others!”. Or as I say, “Committest though NOT the 2nd Sin of Thermodynamics!”. The 2nd sin is to confuse a joule of heat with a joule of work as if they’re of equal value- rather like confusing an American dollar with a Jamaican dollar because they’re both units of money measured in dollars!
I have a device which can convert electricity to 1000 C heat with 100% efficiency! Is that magic? No, the device is called a resistance heater. Converting pure exergy to even high temperature heat with 100% efficiency is no problem, because that heat has a lower exergy value. The 2nd law says, “that’s fine- thou shalt pass”.
On the flipside, one can use a joule of work to PUMP 3 joules of heat from a cold place to a hot place. The 2nd law is OK with that too, with the temperatures of the hot and cold places determining the maximum efficiency (called, for heat pumps, a coefficient of performance).
An electrolyzer is no more magical than- and in fact, in thermodynamic terms, it’s fairly similar to- a resistance heater. It converts pure exergy (electricity) into a fuel, which is chemical potential energy- a proxy for heat. That process is in the 2nd law’s natural direction, the direction of increasing universal entropy and decreasing useful exergy.
This is the fundamental problem with using hydrogen derived from electricity, as a fuel or energy storage medium. Even at high ENERGY efficiency, making hydrogen from electricity is a massive step backward in EXERGY efficiency.
The current state of the art of water electrolyzers is about 83% HHV (ultimate, thermoneutral) efficiency on the stack level. That means to produce 1 kg of H2 with a HHV of 39.4 kWh/kg- the total heat energy produced when you burn that 1 kg again, including the (useless in exergy terms) 6.1 kWh/kg worth of heat of condensation of the product water vapour- you need to put in 39.4/0.83 or 47.4 kWh of electricity.
Of course a hydrogen plant contains much more than just an electrolysis stack- it has “pumps and tanks and sh*t” too- as my old boss used to say about stuff that looks complicated but really is pretty routine. That stuff- compression equipment, hydrogen deoxygenation and drying, electrolyte management, heat rejection, water treatment etc. etc., a) consumes electricity too, b) costs money and c) is already mass produced, so learning curve savings in cost or big improvements in its efficiency are both unlikely. Add in these “balance of plant” and “outside battery limits” energy uses and hydrogen costs somewhere between 50 and 65 kWh/kg to make from water.
The Efficiency Bargain
Recent claims by two firms- Hysata, with a capillary water electrolyzer, and H2Pro with a complex multi-stage semi-batch electrochemical water splitting scheme, are that they can make 1 kg of hydrogen for 39.4/0.95 = 41.5 kWh. That’s a savings of 5.9 kWh/kg. What percent improvement that is, depends on what denominator you choose, and is hence not all that useful.
These new electrolyzers are both a) new, so no learning curve yet to make them cheaper and b) more complex than state of the art electrolyzers. Both are early stage, i.e. low technology readiness level. To know if either will EVER be useful for making hydrogen at scale, we’d need to know lots and lots of things:
- capital cost now, ie. complexity, ease/difficulty of manufacture, raw materials cost etc.
- how the capital intensity per kg of H2 produced might be reduced when production is done at scale (ease of mass production, basic complexity (how simple is it, and can it be made simpler to reduce cost), ability to scale rather than number up units etc.), and threats to the supply chain (availability of catalytic metals is a big risk for PEM units for instance)
- durability, i.e. how many hours on stream the stack or catalysts will last before requiring replacement
- efficiency loss: how quickly the catalysts or other components lose effectiveness and hence drop efficiency
- etc. etc. etc.
Some of these things won’t be known until a unit has been in industrial service for ten years. Some, we can get good guesses at fairly soon, but only if we’re under an NDA with the company so they open their technical kimono and show us the technology away from the investors and stock promoters, warts and all- so no, you’re not going to find that stuff in a media article!
The fundamental bargain here however is really simple: how much extra cost is it worth to save 5.9 kWh/kg H2?
Taking an electrolyzer which is found in working order, free in a ditch somewhere, which magic elves install free of charge on borrowed land, and with a system efficiency of 50 kWh/kg, to make $1.50/kg hydrogen, it would need to be fed electricity at 3 cents per kWh, i.e. $30/MWh. Add any capital cost at all, and this price of electricity must fall even further, or the price of hydrogen must rise to pay back the capital investors, and the non-magic elves who expect a salary, and the people who don’t give away land for free. If the capital is more than zero, then it will also matter how frequently that electricity is available at a low cost. Even if it’s free part of the time, if it’s not cheap most of the time, your electrolyzer is going to make expensive hydrogen.
5.9 kWh x 3 cents = about 18 cents per kg. You’re not going to pay for much additional capital cost with those savings. Mind you, the 50 kWh/kg electrolyzer is already a pretty expensive beast, because it’s running at a low current density to be so efficient. Real ones you can afford are going to be closer to 60 kWh/kg on a system basis. But who knows what current density Hysata and H2Pro’s claims are made at? I wouldn’t trust the figure even if I could find it in print. And we’ve already established that the capital cost will be higher, because these systems are more complex than the current state of the art- in one case because of complex microstructure (capillaries) and in the other, because it’s a sequential semibatch operation, with oxygen evolution happening separately from hydrogen generation. That takes more “tanks and pumps and valves and sh*t”…
So: are Hysata or H2Pro “breakthroughs” that will revolutionize hydrogen production? Doubtful. They may, or may not, marginally help. But they don’t fix the fundamental problem with electrolysis- they don’t stop it from being, fundamentally, a massive destroyer of exergy.
Steam Electrolysis: SOECs
If you electrolyze steam rather than water, you can actually make hydrogen for even less than 39.4 kWh/kg input electrical energy. You can actually take some heat and convert it to hydrogen chemical potential energy. Of course the device is a stack of metals and ceramics glued together with glass, which operates at around 700 C, so you’re not getting off scot free here. Even if you can find somebody who will boil water for you for free, rather than paying another 6.1 kWh/kg H2 to do that, you have the problem of running at 700 C. And given that 7/8 of the mass coming out of the electrolyzer is oxygen, recovering heat from what amounts to a cutting torch is, well, tricky. SOEC stacks are small, don’t like thermal cycling so have to be electrically heated to keep them hot when they’re not operating, and today cost considerably more per MW of capacity than water electrolyzers- for reasons that should be obvious. Are SOECs a magical solution? Depends- does your process need both hot oxygen and hot hydrogen? Then, maybe. Otherwise, doubtful in my view, unless somebody makes a huge leap forward in making SOEC run efficiently several hundred degrees cooler.
Cycle Efficiency
The problems with hydrogen as a fuel or energy carrier show up when you do a cycle- from electricity to hydrogen, hydrogen to storage, and storage to a fuelcell or turbine or other device to make electricity again.
Of course the ridiculous commentators say that the cycle is 100% efficient! And they’re right- it’s 100% efficient at turning electricity into heat, most of which is worthless. Sadly, a chunk of heat (electrolysis waste heat, and compression or liquefaction waste heat, or ammonia/methanol/LOHC hydrogenation/e-LNG waste heat, is made where you already have energy in excess (and hence are throwing away electricity). So yeah, that waste heat is GONE- it rarely has a meaningful use, except perhaps in complex process units i.e. with the waste heat used to run the Rube Goldberg apparatus known as direct air capture or the like. Affording the capital to recover this heat is a different matter, and a value proposition there is absolutely NOT guaranteed. The smaller the scale, the less likely that source heat recovery will be useful.
Using best case figures: electrolysis at 83% HHV efficiency is really about 70% LHV efficiency (subtracting the 6.1 kWh/kg of heat energy which no fuelcell or engine can put to use). Best case, compression for storage is about 90% efficient, at scale- at smaller scale, this efficiency falls rapidly. And the best case for either a fuelcell or combined cycle gas turbine power plant is about 60% on a LHV basis. 0.7 x 0.9 x 0.6 is 37%, i.e. buy 3 kWh, get 1 back. That’s just fundamentally a terrible battery- even worse, because the energy density of hydrogen per unit volume is absolutely terrible. And on top of that craptastic efficiency, you end up needing to pay for a bunch of expensive capital equipment. Note there’s nothing in that simple calc for transmission of electricity, transmission of hydrogen product, or innumerable other energy-sucking factors in real systems. It’s an idealized best case. And no, changing that 70% LHV efficiency to 80% (i.e. 95% HHV efficiency) doesn’t help all that much- the cycle increases to 43%. On the same basis, a lithium (or sodium) ion battery is 90%. And that battery is very, very much simpler.
A nice visual example, because people like pictures: here’s David Cebon‘s graphical explanation of why it is so much more sensible to use the exergy of wind electricity to pump heat into homes, than to use it to make hydrogen to replace fossil gas in existing boilers. There we’re talking about a ~ 5-fold difference in useful energy delivered per joule of feed energy.
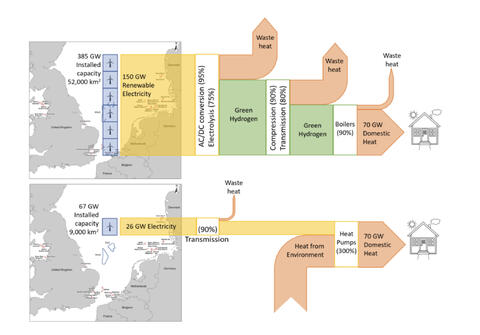
Poor cycle efficiency is what kills hydrogen’s use as a fuel, whether for transport or heating or what have you. Poor efficiency which is NOT offset by much greater effectiveness (i.e. ease of storage, transport or use of energy) is just a recipe for economic failure. It’s putting money into two piles on the floor and setting one of them on fire. That might make you money under some rare circumstances, but generally only if the money you’re burning, isn’t yours!
Disclaimer: I’m human and so I make mistakes. I don’t know everything, either. Where I’ve gone wrong, provide me with good references to the contrary and I’ll amend my text with gratitude. Don’t like it because I’m slaughtering your precious idea with my analysis? Feel free to complain to Spitfire Research Inc., who will tell you to shove off and write your own article.