This article is a summary of my series of posts about electric heating:
https://www.linkedin.com/posts/paul-martin-195763b_electric-heatingfinal-thoughts-its-clear-activity-7236112474733735936-MXfw?utm_source=share&utm_medium=member_desktop
Some people have it in their head that there’s no way to make heat other than via fire. The reality is more complex. The reason we use fire to make heat is that not only do we have an 800,000 year history doing just that, but also because if you can find burnable fossils lying about in the earth, and have a free atmosphere into which you can dump the effluent, burning stuff is the cheapest way to make heat- under most circumstances.
Note that we make heat from electricity today, despite its higher cost, in lots of applications where fire doesn’t do the trick, i.e. fire is ineffective for some reason or another. And in a world where we make electricity largely from heat, i.e. by burning stuff, using electricity to make heat is pretty crazy- it would need to provide a rather huge effectiveness benefit to overcome all the inefficiencies of making a fuel, transmitting it, converting it to heat, then converting that heat to a (much) smaller portion of electricity which is then converted to…heat.
Those basic assumptions have changed though. If you don’t think they have, then please just go away, as you’ll bring nothing to the conversation.
In a decarbonized future we’ll be starting with electricity, largely from wind and solar, but perhaps also from nuclear or out of storage. And in that case, converting electricity partially to something to burn as a way to make heat, is similarly insane. We’ll do that only if we’re both very rich and very desperate.
Residential and Commercial Heating
People in northern climates need to heat their homes. This isn’t for mere “comfort”, it’s a necessity of life.
Most of us still heat with fire here in Ontario, myself included. That’s because historically, gas was so very cheap, especially relative to retail electricity. And most of us consider the resulting GHG emissions to be worrisome- even though residential heating represents shockingly only 5.5% of Canada’s GHG emissions on an annual basis. That’s not because our climate isn’t cold, or because we’re hyper-efficient with our heating. Rather, it’s because we generate vastly greater emissions in other sectors of our economy, especially in transport. That likely surprises people more than it surprised me back in 2019 when I first saw these figures.
I’ve taken the time to update my 2019 article about residential heating electrification, using my own Toronto home and its energy use and costs as the reference case for comparisons.
https://www.linkedin.com/pulse/home-heating-electrification-paul-martin
The 2018-based figures used in my 2019 analysis showed that heating electrification produced quite high costs per tonne of CO2e emissions averted, even taking rather high estimates of methane leakage into consideration at the 20 year global warming potential of 84x CO2. However, my updated analysis using 2022/2023 data indicates that you might even save money if you were smart about using an air-sourced heatpump in Toronto. That’s despite the fact that the 2023 carbon tax rate was only $65 CDN/tonne CO2e on natural gas- it’s $80/tonne in 2024, making the opportunity even more compelling.
The key technology here is heat pumping, which allows us to use electricity as exergy, i.e. thermodynamic work, rather than grinding it up into heat in a resistance heater. Heat pumps allow us to use 1 joule of electricity to pump, on a seasonal basis, perhaps as many as 3 joules of heat from the cold air outside, into our homes- or as many as 5 joules of heat from soil and groundwater if we have the money to afford drilling wells to access that heat. And before the Albertans and Saskquatches jump on me, I’d like to remind readers that 80% of Canadians a) live in urban rather than rural settings, and b) live within 100 miles of the US border. So while it will be harder to justify heat pumping in Edmonton, much less Fort McMurray, relative to warm-ish Toronto, it’s still an option within reach of most Canadians. My brother in Alberta proved that by doing it in his home, and I’m very proud that he beat me to it!
And no, hydrogen as a home or commercial heating strategy, isn’t going to be a thing, regardless how hard the natural gas industry pumps the idea to stay in business- or at least to pretend to have a future post decarbonization.
Industrial Heat Pumping: Distillation
Somewhere between 40 and 60% of industrial heat is consumed below about 200 C.
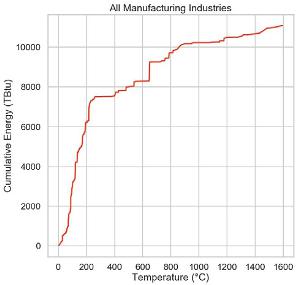
Much of that heat today is produced by burning fuels, often transferred by means of steam. Steam is generated from combustion and sometimes from waste heat from other processes, just as a means to move heat around conveniently.
My future article about electric heating will provide more detail and some sketches to illustrate things more clearly, but for now, let’s look at a major use of industrial heat: distillation.
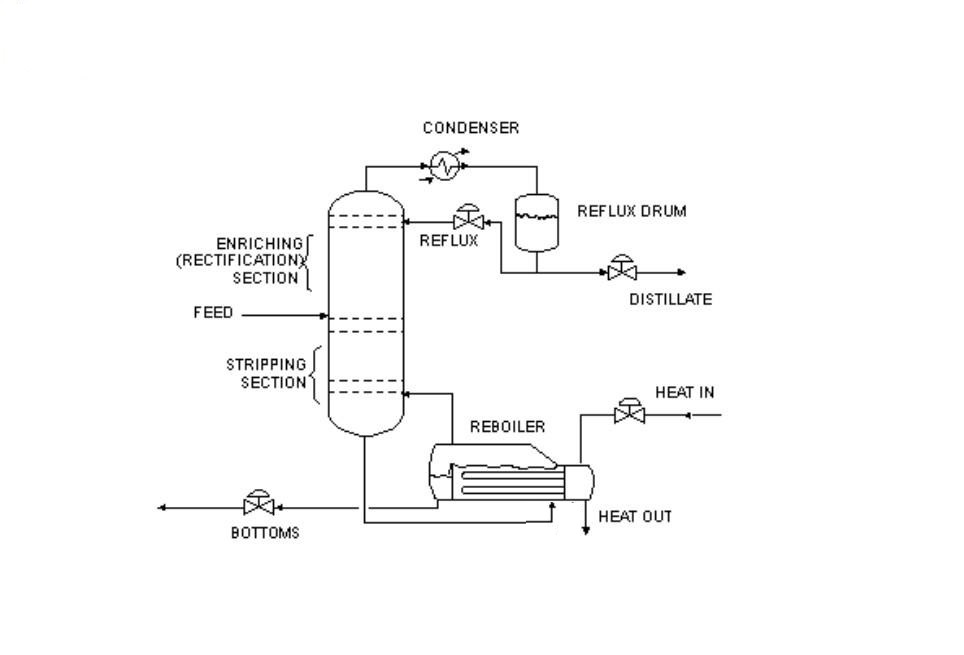
Commercially, most distillations are continuous, not done in batch. A liquid mixture is fed continuously to the middle of a distillation column, which has trays or packing which allow rising vapour to intimately contact with falling liquid. A condenser at the top of the column, condenses some or all of the vapour and allows the liquid condensate to flow back down through the packing (referred to as reflux). A re-boiler at the bottom of the column, generates vapour from the liquid which leaves the bottom of the column. Compounds in the mixture separate by their “relative volatility”- which depends on lots of stuff, but the most important thing is their relative boiling points. Higher boiling liquids tend to concentrate in the bottom of the column, and lower boilers near the top. Liquid products can be taken off the column continuously at any point where the composition is what you want- off the condenser, off the reboiler, or off various points along the column.
Normally, the reboiler is fed a hot medium- steam, a hot liquid, or sometimes, flue gas from fuel burning. And the condenser is cooled using a cooling medium- cooling water, air, or sometimes, another stream that needs to be heated up. Nearly every joule fed to the reboiler, comes back out of the condenser- just at a lower temperature.
We can electrify distillation by the simpleminded method where we use a resistance heater in place of the reboiler, but that would be wasteful.
We could instead use a normal refrigerant heat pump to pump heat from the condenser, back into the reboiler. The smaller the temperature difference between the reboiler and the condenser, the easier it would be to pump heat from the condenser back to the reboiler, and the higher the coefficient of performance (the less electricity we’d need to pump the required amount of heat).
But if we are permitted, we could instead simply take the vapour off the top of the column and feed it to a compressor directly. The vapour would leave the compressor “superheated”, and would enter the tubes of the reboiler where it would condense at a higher pressure, providing heat to boil the fluid in the reboiler. This scheme, called “mechanical vapour recompression”, uses the process vapour as the refrigerant, which not only improves the coefficient of performance, but also eliminates at least one heat exchanger entirely. There’s no separate condenser and reboiler any more- one unit does both jobs. A small liquid subcooler might be required to prevent the reflux or distillate from flashing as it exits the reflux drum, but this would have a very small duty relative to what the condenser previously had.
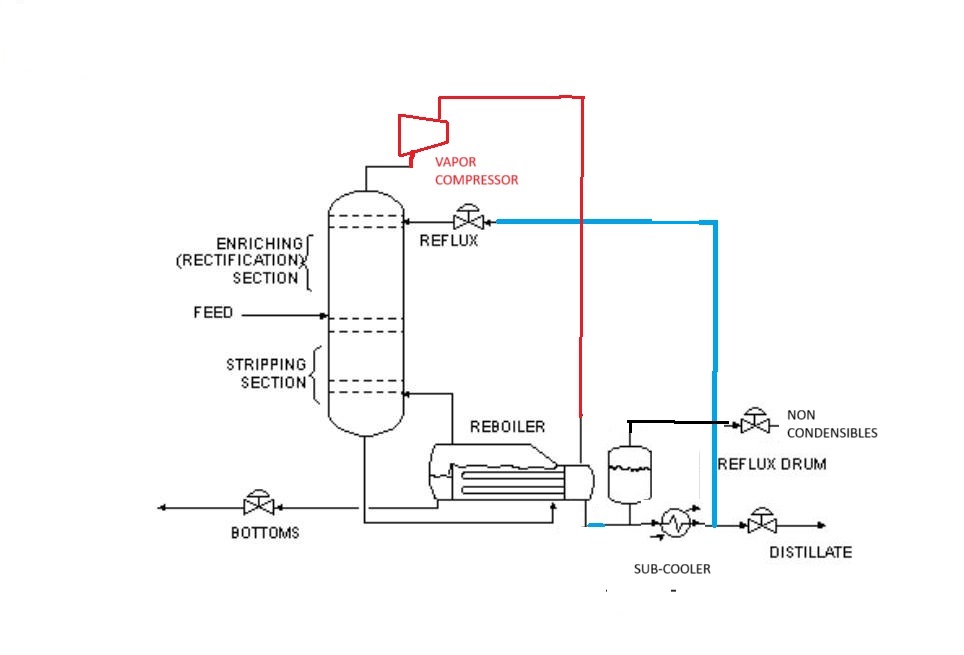
Medium Temperature Industrial Heating- 200 to 800 C – Resistance Heaters
A smaller fraction of industrial heating requires heat at higher temperatures, above 200 C, where heat pumping schemes are presently impractical. These are harder to afford, because none of the approaches feasible in this temperature range are capable of converting 1 joule of electricity into more than 1 joule of the required heat. Burning up pure exergy (electricity) to make heat is of course dead easy to do technically, but not easy to afford!
The conventional approach within this temperature range is to use resistance heaters, which are as simple as they sound: electrical resistors which heat up when you pass a current through them.
In practice, resistance heaters for temperatures below 800 C (and in fact for temperatures above this range under certain conditions) all look more or less the same: they consist of a coil of resistance wire made out of a high temperature metal alloy. That coil of wire is either immersed directly in air or another gas (examples: a hair dryer or toaster, or a ceramic kiln), or it is protected from the thing it’s heating by a sheath.
Bare wire heaters have the advantage of direct contact with the thing being heated, if that thing is a gas. They can be very cheap indeed. They can also be heated to very high temperature such that their heat transfer is via radiation (infrared light) more than convection- that’s what’s largely happening in your toaster for instance. They also have the distinct disadvantage of…direct contact with the thing being heated. This can lead to:
- Corrosion, causing the wire to get thinner and hence higher in resistance, which causes it to get thinner…leading to an autocatalytic failure
- Deposits may form on the wire, which can insulate the wire from the thing being heated, allowing the wire’s local temperature to rise high enough to cause a mechanical and then an electrical failure of the wire
- Decomposition of the thing flowing by the wire, if it isn’t totally inert to the wire material, or if it is sensitive to over-temperature. This can cause product degradation
They also suffer from very low surface area as you can imagine. Since heat flow is proportional to the area of the hot thing, higher temperatures are required for a small thing to emit the same heat as a large thing. Longer wires are therefore required if you want the wire temperature to be kept low- or, if you’re at such a high temperature already that any higher temperature will cause the wire to rapidly sag (creep) and fail.
They also obviously cannot be used to heat media that are themselves conductive to electricity- otherwise, the thing carrying the current will not be the wire, but the thing you’re trying to heat…Sometimes, this approach, called “ohmic heating”, i.e. passing the current directly through the thing you want to heat up, is used- but we’ll talk about that in another article.
Immersion Heaters
In my previous post, I talked about bare wire heaters such as those used in a ceramic kiln or a toaster. In this post I’ll talk about immersion heaters, which are the workhorse of electric heating in the process industry.
Every chemical engineer is familiar with the shell and tube heat exchanger. A fluid, hot or cold, flows through a number of tubes that pass through a shell through which another fluid flows. Heat is transferred through the tubes to the fluid in the shell, or vice versa. There are numerous arrangements of S&T exchangers, each best suited to a particular set of use cases.
In principle, an electric immersion heater is just a shell and tube heat exchanger whose tubes have electrons rather than a hot fluid flowing through them. The tubes heat up, and fluid passing by the tubes in the shell, receive this heat by convection and radiation.
Each tube consists of a wire heater inserted into a tube which is then packed generally with magnesium oxide, which has the properties of inertness and decent thermal conductivity while having low electrical conductivity even at very high temperatures.
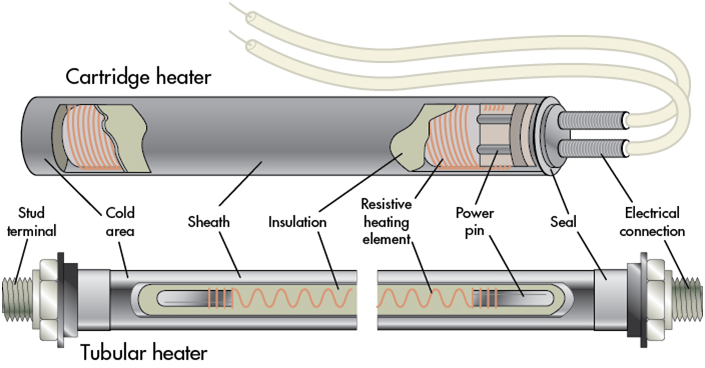
The tube is generally then bent into a U shape like a hairpin, so that both ends of the wire (where the electrical connections are made) are at one end of the assembly. The U shaped tubes are inserted through a flange to which they are either welded or brazed. Heaters can have one or thousands of such Us on a common flange. The result is that we now have basically a “BEU” heat exchanger bundle, except the bonnet is where the electrical connections are made.
The elements are then wired in the bonnet with bus bars, in combinations of series and parallel, to provide the required resistance at the supplied voltage to obtain the necessary heating power.
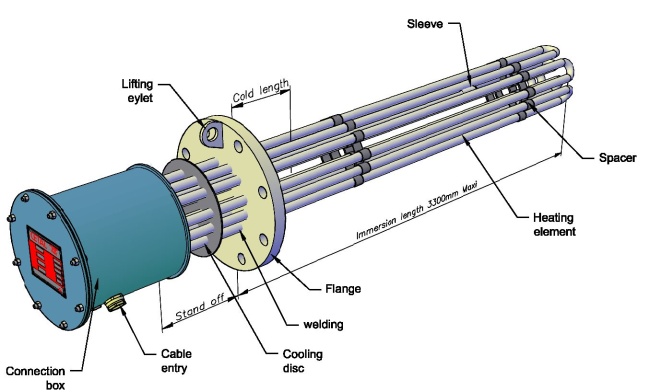
Each flanged heater is inserted into the end of a shell. Generally, either one or two flanged heaters are used per shell.
Unlike with S&T heat exchangers, there aren’t generally baffles along the tube bundle. Flow through the shell is therefore not serpentine, but annular. Why are baffles not used? Because they tend to accumulate deposits or serve as corrosion initiation points, and lead to premature failure.
You can see the advantages of the approach:
- The wires are protected from the flowing fluid by a metal tube, which can be made of whatever alloy is resistant to the flowing fluid
- The wire is supported by the MgO insulation, so it can’t sag
- The flange and tubes are designed for whatever pressure you need- we’ve had heaters made up to 2500# flange class
The major disadvantage of course is that now the wire has to get hot enough to heat the MgO sufficiently to heat the tube to the temperature needed to transfer the required heat. And if your process temperature is very high, you might soon exceed the limits of the wire, even well supported by the MgO.
We’ve had immersion heaters of this design, built to heat gases up to 850 C continuously, and they have provided excellent service- because we knew what we were doing in designing, controlling and operating the system. But at those temperatures, nobody can guarantee how long such a heater will last. Failure is only a matter of time, though there is much you can do to prolong their life.
Very High Temperatures
As an engineer who spent most of his career designing equipment to push the limits of chemical process technology, one thing that I couldn’t miss is the fact that the safe allowable stress values for all metallic materials and alloys, basically drop off a cliff around 800 C, limiting their ability to be used to support things or resist pressure. The reason for this is “creep”, the tendency of alloys to change their shape over time even under small amounts of stress.
We contend with this problem in industry in several ways. One is to manipulate conditions so that the mean temperature of the metal is kept to a reasonable level by playing games with heat transfer resistances. This is how an ordinary steel tube can be kept safe in a heat recovery steam generator, when one face of the tube is exposed to very hot gas or even to a flame.
Another is to keep the supporting metal cool by using a refractory material on the hot side. The refractory can be “hard”, to resist erosion and other wear and tear, or “insulating”, or a compromise. The cold side must remain exposed or in some cases, must be actively cooled.
And a third, when we need to transfer heat at very high temperatures, is to use tubes made of superalloys and just realize that they will eventually fail due to creep and need replacement. That’s the strategy used in steam reformers, where the tubes operate at temperatures well beyond the limits of the normal design codes for pressure equipment. Failing tubes are literally pinched off inside the furnace while the unit continues to operate.
A key limiting factor for electric heating therefore is the strength of materials at high temperatures. And there are several solutions:
- Use superalloys: FeCrAl alloys (i.e. tradename Kanthal) are king here, but they are difficult to fabricate. They are among the best, most durable choices for heating wires used inside heating elements, but it’s tough to make a whole heat exchanger out of FeCrAl
- Use refractory metals: molybdenum is the frequently reached for material here, but it too has serious issues other than just its cost. The elements, after the first heating, become brittle
- Use nonmetals: heating elements can be made of silicon carbide, molybdenum disilicide, graphite and other nonmetallic materials. Each of these has its own upper temperature limit, and a set of conditions which kill them
The last option is to switch to another method of electric heating- the subject of future posts.
Other Kinds of Electric Heating
I could write a book on the subject of electric heating, based on my couple decades of doing it for pilot and demonstration scale plants of all sorts. But even I rarely read books any more, because by the time they’re published, things have already changed! So if you really want my advice about these issues, you are best advised to visit www.spitfireresearch.com and find out about my consulting services.
We’ve talked about heat pumping and resistive heating at some length. Now I’ll gloss over a number of other ways to use electricity to heat stuff, spread over a couple posts.
Induction: conductive things like metals and graphite can be heated by inducing eddy current flow in them using a varying magnetic field. This is commonly done to rapidly heat piece of metal to temperatures suitable for forging, for instance, but it can be applied to heating all sorts of things. It has become a very popular replacement for gas for cooking, where it is greatly more energy efficient and lower in emissions. There are some losses, because the conductor producing the magnetic field has its own ohmic losses and these need to be removed (often via cooling water) The switchgear needed to generate the induction current also represents some losses- moreso than in resistive heaters.
Microwave and RF Heating: microwaves and radio-frequency radiation can be used to excite certain molecules or parts thereof directly- particularly stretches and rotations of O-H bonds in water, sugars and the like, resulting in heat generation. Heat transfer is therefore not from the surface inward, but from some depth both inward and outward, the depth depending on how strongly the radiation is absorbed. It is sometimes possible to accomplish the desired result (a chemical transformation, drying etc.) without heating the bulk of the material to the same uniformly high temperature, resulting in very real energy savings relative to convective or conductive heating.
Microwaves are fairly inefficient as electric heating goes, with about 20% of the fed energy being lost as heat in the apparatus. Microwave emitters are also limited in maximum power to around 100 kW, requiring “numbering up” of smaller apparatus which can be a real drag on economics at scale.
Mechanical Heating: viscous materials such as plastics, which have poor thermal conductivity and a limited maximum temperature making resistive heating a real problem, are frequently heated by vigorous mixing using an extruder. In this case, work done on the material becomes frictional heat delivered to the bulk of the material. That seems insane at first blush- producing heat by friction! But remember, we’re starting here with work– converting it to heat in a resistor is no less crazy.
There are other methods of producing heat, sometimes of very high temperature, by doing work on a gas, producing shock waves etc. These are so far experimental without full-scale demonstrations as far as I’m aware, but they may be useful for some gas-phase reactions currently heated using tubes in furnaces.
Replacing Fire
The really hard applications for electric heating are applications where heat transfer through a conductive heat transfer surface won’t do the job. That can happen because the conductive materials we might want to use are not strong enough at the high temperatures required. An example is the interior of a cement clinkering kiln. There, we use a rotating steel tube which is lined inside with refractory bricks, and we put a giant radiative fire in the centre. Heat is transferred from the fire to the solids by radiation, from the flue gas to the solid by convection, and from the fire to the bricks above the solid which are then rotated into contact with the solid. Such a flame needs to be powerfully radiant though, so hydrogen’s a poor choice. In fact even gas isn’t perfect- glowing particles of burning coal dust are the best.
Fortunately, we aren’t out of tools in the electric heating toolbag.
We can in fact make “flames” with electricity and just about any gas, including air. Flames are just chemically heated plasma- a soup of ions and electrons- which radiate light (from UV to IR inclusive, depending on temperature). We use electrically heated plasmas to weld, to cut metal, and to heat gases to very high temperatures. Plasma arcs are complicated- they can be “cold” (i.e. corona discharge, used to make ozone from oxygen) or “thermal”. They can be struck between electrodes, or generated (under vacuum) by microwave excitation etc. They can be steered and focused by magnetic fields. They can be excited by AC or DC currents. And the gas they turn into a plasma can be air, or it can be vapour from the electrodes or the thing being heated.
Temperatures of 30,000 C can be achieved. But of course many things determine whether or not a plasma arc is a practical way to heat your particular thing. Very many, complex things…far beyond our scope here.
A example is the electric arc furnace (EAF). About 70% of steel in the USA is made using EAFs. A charge of scrap mixed with some fresh iron is fed into a refractory lined ladle, into which huge carbon electrodes are fed. The electrodes carry current which contacts the scrap and heats it by resistance heating, but there is also a plasma arc struck between the electrodes and the steel- and each other. The electrodes evaporate and react with water and oxygen which might be present in the scrap, so they are continuously consumed. CO2 emissions therefore occur- but some of the carbon also ends up where it’s needed, in the iron, to make steel. There are other applications of arc melting, including melting ceramics or steelmaking slag to spin ceramic fibres.
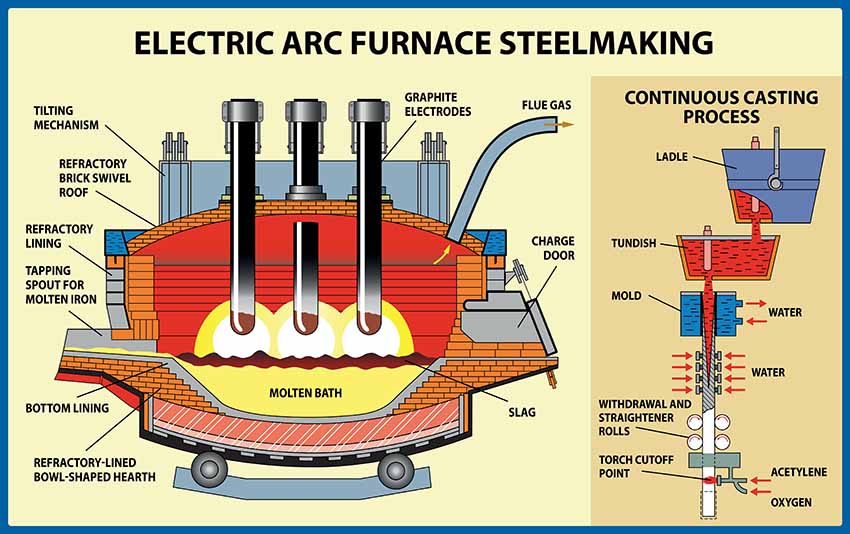
Finally, direct ohmic heating is sometimes possible under conditions that you wouldn’t at first think possible. Molten glass, for instance, has sufficient ion mobility that it is electrically conductive. Molybdenum electrodes inserted into the molten glass can be excited with AC currents to heat the molten glass to the required temperature for forming. Ohmic heating doesn’t consume the electrodes- at least not so quickly that they need to be continuously fed- and doesn’t involve a plasma arc.
Electric Heating: Final Thoughts
It’s clear that most people still have a burner box on their heads about electric heating particularly in industrial applications, which is no surprise as we humans have been burning stuff to make heat for about the past 800,000 years.
Let’s be clear: with precious few exceptions, this isn’t about a lack of a technology, or a need for fire. It’s about a lack of economic drivers- durable carbon taxes and emission bans. Without those, fire is just cheaper, and electric heating is used only where it provides greater effectiveness, i.e. convenience or cleanliness or ease of control. That’s electric heating today, in a nutshell. But that is not electric heating’s future!
It’s clear that residential and commercial heating is transitioning to heat pumps. Whether hybrid heat pumps with a supplementary fuel source for the very coldest days are worth thinking about, depends on how cold it is where you live, and how clean your grid might be.
Industrial heating below 200 C, where most industrial heat joules are actually used, is similarly going to heat pumps- but the heat source won’t be ambient air or groundwater. It’ll be waste heat from inside these facilities. Two birds with one stone- this not only cuts GHG and toxic emissions from fuel combustion, but also greatly reduce waste heat emissions to rivers and lakes, and freshwater loss through evaporation in cooing towers. We can stretch this to 300 C if we try hard enough! And yes, you CAN make steam with a heatpump- if your cold reservoir is hot enough.
Conventional heat recovery schemes rely on taking heat from fire and letting it trickle down the temperature range, passing from stream to stream on the way down. We’ll keep doing that for temperatures above 300 C, and will be encouraged to do more of it as the cost per joule of heat increases- as a switch away from fire to resistance or other high temperature heaters will necessarily do. In the future, below about 300 C, we’ll likely recycle a lot more heat via heat pumping, which will stand current heat recovery practice on its head.
Higher temperature heating is economically harder because efficiency is “only” about 100%. But we have lots of tools in our toolbox for industrial uses, even to temperatures simply impossible with fire.
We know how to heat stuff with electricity. The only question is, will we be smart enough to do so?
Finally: Hydrogen For Industrial Heating
There are a few places in industry where flames and/or hot flue gas are really needed. While hydrogen might be an option for some of these applications, the lack of a radiant flame, the tendency of hydrogen combustion in air to make NOx (just as burning anything else in air does), and the cost of hydrogen derived from electricity, make this an unlikely choice. Biogas methane makes more sense, as do some other biofuels. Most of the thinking around hydrogen as a natural gas replacement are just simpleminded fuel substitution thinking- the thermodynamic equivalent of “let them eat cake”. And no, hydrogen blending into the natural gas network, isn’t going to be a thing either. This article’s title is rude, but that’s for a reason- the idea really is bollocks.
https://www.linkedin.com/pulse/why-hydrogen-blending-gas-network-bollocks-paul-martin-i5sdc
Disclaimer: This article was written by a human, and humans are known to make mistakes from time to time. If you find an error in what I’ve written, and can demonstrate that with good references, please make a comment and I’ll be happy to correct my work.
If however you don’t like what I’ve written because it takes a dump on your pet idea- hydrogen for heating for instance- then I encourage you to contact my employer, Spitfire Research Inc., which will be happy to tell you to piss off and write your own article.