The Primary Energy Fallacy- or, Committest Thou NOT the 2nd Sin of Thermodynamics!
TL&DR Summary: if anybody starts talking to you about “primary energy” in a discussion about decarbonization, please punch them in the mouth. Energy is like currency- there’s an exchange rate between heat (chemical energy) and work (electricity)- they are not worth the same. We need to replace useful energy- energy services- not rejected energy arising from heat to work conversions. Decarbonization is therefore ½ to 1/3 as difficult as these cretins would have you believe.
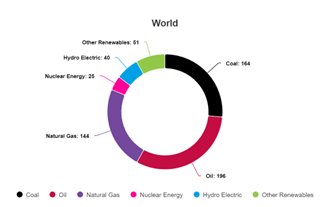
Source: https://www.energyinst.org/statistical-review Primary energy figures, worldwide, 2023
We’ve all seen graphs like this before, from people claiming that the world’s “primary energy” almost entirely (per this one, 84% in 2023) comes from burning fossils as fuels. And we see these graphs barely changing with respect to time too. The likes of Canadian professor Vaclav Smil have, in past, been popular proponents of the argument that a transition away from burning fossils as a source of energy is basically next to impossible, or so impractical that it simply won’t happen in less than 100 years, because of primary energy use and our seemingly insatiable demand for oil and gas- not so much coal any more, but hey, they still burn a lot in China…
These people are wrong. Dead wrong. Sometimes they even know they’re wrong, but often it’s a result of a bad education in physics.
Energy is like money. While we like some units better than others in relation to some kinds of energy, ultimately all energy of all kinds is quantified in units like joules, kilowatt-hours, or British Thermal Units (BTUs). But like money, knowing that I have 100 dollars is not sufficient to know how much value I have in my hand. We need more information: in the case of currency, we need to know if these are American dollars or Jamaican dollars for instance. Both are units of money measured in dollars, but they are not worth the same.
With energy, we’re missing a conversion factor too. 100 joules of heat in a room at 24 C is exactly the same amount of energy as 100 joules of electricity. But whereas that 100 J of heat at just above room temperature isn’t just worthless, it’s actually an energetic liability if we need the room cooler than that, the 100 J of electricity can be nearly perfectly converted into mechanical energy or perfectly converted into heat at just about any temperature we wish.
Exergy- and no, that’s NOT a Typo!
To a thermodynamicist, the term “exergy” has a more complex definition, but to a layperson or the average engineer, exergy is simply the potential of a unit of energy to do thermodynamic work, i.e. to be converted into mechanical energy. 100 J of electricity is pure exergy. 100 J of room temperature heat has an exergy value of basically zero.
The exergy value of heat, or proxies for heat such as fuels, varies in accordance with the 2nd Law of Thermodynamics. Under the 2nd Law, heat engines that convert heat energy into thermodynamic work, have an ultimate efficiency of conversion which is related to the temperature of the “hot reservoir” (the source of heat) and the “cold reservoir” (the place the heat is ultimately being rejected to). You can think of this as basically a waterfall. Heat is flowing from the hot place to the cold place, which is the only direction it flows spontaneously. And we’re running a device on the difference in temperature- a waterwheel of sorts, harnessing some of the flow of heat in its natural flow direction.
The efficiency reaches a limit set by the Carnot efficiency, which is the ratio of the available temperature difference to the temperature of the cold reservoir, in absolute temperature units (degrees Kelvin, i.e. relative to absolute zero at 0 K).
Some devices for converting chemical energy to work aren’t heat engines per se. A fuelcell, for instance, converts chemical potential energy into electrical energy without any moving parts. No mechanical energy is involved. However, all such devices have their own set of thermodynamic and other practical limits which drop their efficiency below 100%. The most efficient fuelcell is at present about as efficient as the most efficient full scale heat engine, a combined cycle (gas turbine + steam turbine) natural gas powerplant. Both convert about 60% of the lower heating value of their feed fuel, to mechanical energy. And unless the fuel is pure carbon, the fact that we’ve used the lower heating value of the fuel, basically means that we’re throwing away the difference between the lower (net) and higher (gross) heating value of the fuel, which corresponds to the heat required to condense any water vapour produced by combustion and to cool that water down to room temperature. That energy is not available to the heat engine to use, but if we were running the reverse reaction- making hydrogen as a fuel out of liquid water by electrolysis, for instance- we’d have to put that energy IN to satisfy the 1st law of thermodynamics- the conservation of energy.
We’ve gotten increasingly good at converting heat energy into work.
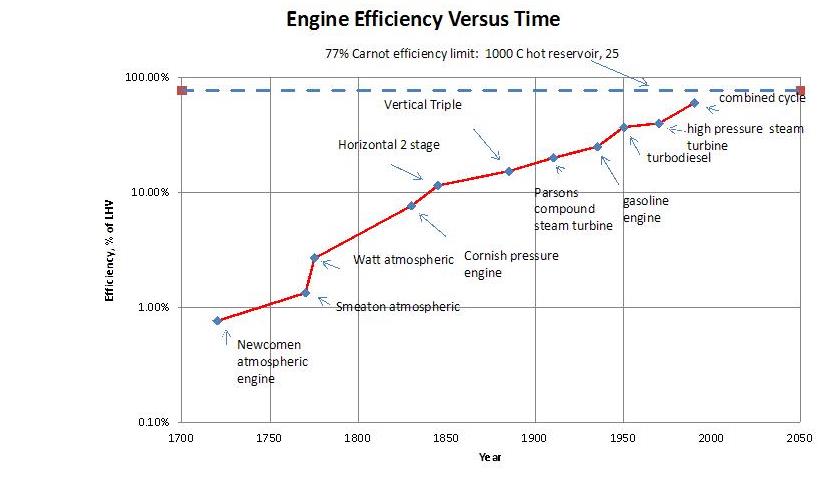
And we will indeed need to make some heat into work in a decarbonized future. We’ll still have geothermal power plants, and nuclear power plants, which work this way. But today, because we make ~84% of our “primary energy” from chemical energy in the form of fossil fuels, we convert an awful lot of heat to work. And, per the 2nd law, we do so very inefficiently. Most of that energy isn’t fed to 60% LHV efficient combined cycle power plants. Much of it is fed instead to much lower efficiency engines such as those in the average car or truck, with perhaps a 25% efficiency of converting chemical energy into shaft work. The remaining 75% is just wasted.
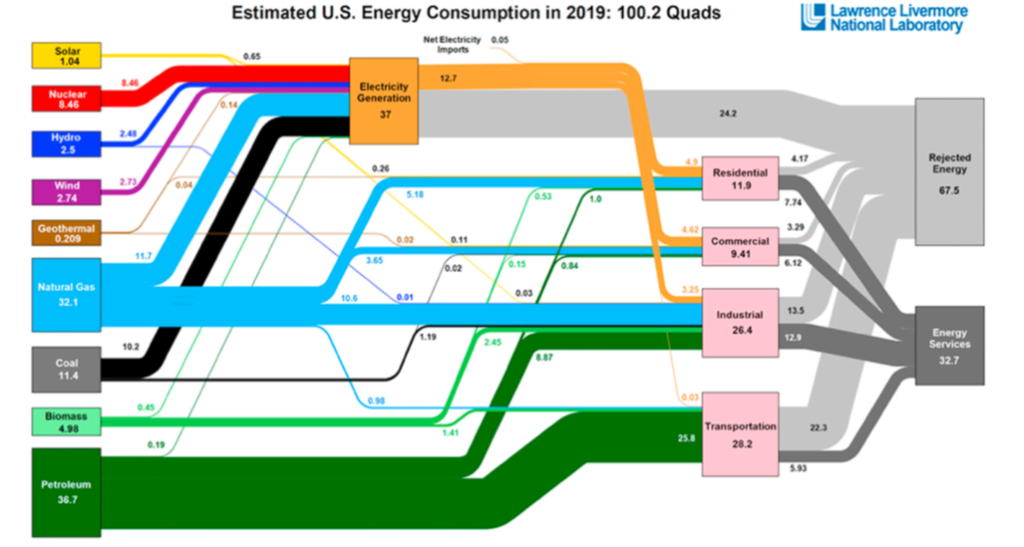
That’s the very large, ugly, light gray coloured box in this Lawerence Livermore National Laboratories Sankey diagram of US energy flows, labeled “rejected energy”. And most of that light gray box is going away in a decarbonized future. We simply do not need to replace that rejected energy.
Why not? Because in a decarbonized future, we’ll be starting with electricity- which is nearly pure exergy. And if we’re smart, we’ll use that electricity in high efficiency processes to produce the “energy services” (the dark gray box) that we need.
The most obvious of these is that we will no longer be burning fuels to convert a small fraction of that fuel energy into electricity. But the near elimination of rejected energy doesn’t end there, not by a long shot!
Electrifying Transport- Getting Rid of Rejected Energy
The easiest case in point is the electrification of transport. Most transport today uses fueled internal combustion engines, and most of these engines are very inefficient, wasting most of the chemical potential energy they are fed.
Battery electric vehicles, in contrast, are very much more efficient. Lithium ion batteries, including their charging circuitry and all their internal losses, are as much as 90% efficient in vehicular applications. And electric motors and the inverters to drive them are also high 90% each. The result is that very few kWh of electricity fed to a battery, end up wasted. Most of the fed electrical energy goes into moving the vehicle forward against rolling and aerodynamic resistance. Even better, a good fraction of the energy normally wasted in an engine vehicle every time you need to slow it down by applying the brakes, can simply be converted back to electricity and stored in the battery again with high efficiency. This is referred to as “regenerative braking”, and it results in an approximately 15% improvement in range while also nearly eliminating the wear on mechanical brakes.
While we won’t soon eliminate fuels from transoceanic shipping and aviation, basically all land transport is going electric. The result is that most of every barrel of petroleum that we use today- 75-85% of which we generally burn for one purpose or another- will no longer be wasted in this profligate way. We’ll be able to conserve that precious, finite fossil resource for its highest value use- to make chemicals and materials. And no, there is no imperative whatsoever to burn anything in that process.
https://www.linkedin.com/pulse/refinery-future-thought-experiment-paul-martin-4pfoc
What will we use for aviation and shipping, and for remote and rural transport? Biofuels- or, in the meantime, fossil fuels. These applications will only shift as a result of durable carbon taxes and emission bans. Over the very, very long term, perhaps electrification will handle shipping and aviation. The former, requires batteries to drop in cost by an order of magnitude, so that cycling them only every 20 days or so will make economic sense relative to burning a fuel- sodium ion may yet do that for us. The latter, will require energy density in batteries to improve by at least half an order of magnitude. That requires a new type of battery-perhaps metal-air. Neither are impossible, but both are at least a decade or two off. There is more than enough biofuels potential to handle both in the meantime, if we feel that is a good use of our treasure. In the meantime however, we should focus on the easy to decarbonize sectors- and that includes almost all of land transport. EVs there are a no brainer.
Heat Pumps: The 2nd Law Standing on its Head
The cool thing about thermodynamics is that it is often reversible. Heat pumps are an example. Where we lose in converting heat to work, we gain in using work to pump heat.
There’s nothing mysterious about a heat pump. The 2nd Law says that heat flows spontaneously only from hot to cold, and we know that to be true because of the kinetic molecular theory. Heat is, after all, vibrating, translating and rotating molecules, bashing into one another. That was the piece of the puzzle that people like Count Rumford and Carnot were missing when they thought of heat.
However, thermodynamics also makes it clear that we can pump heat from a cold place to a hot place by expending thermodynamic work. The example is your refrigerator or air conditioner, where heat is absorbed by an evaporating liquid refrigerant, which is then compressed by a mechanical compressor and condensed again, rejecting heat to air pushed by a fan. Heat flows out of your beer into your kitchen, or out of your house to the outdoors. Nothing magic about it. The maximum amount of heat you can pump with a unit of mechanical energy is similarly set by the temperature difference between the hot place and the cold place.
A heat pump can be used as a heating appliance, taking heat out of ambient air, soil or groundwater, or better still, from something we want to cool down, and transferring it to something we want to heat up. In a typical heat pump used to heat a home, if you feed 1 joule or kWh of electrical energy to the compressor, you can move on average around three joules or kWh of heat from the cold place to the hot place. That includes the 1 joule or kWh of electricity you fed, which is itself converted into heat which flows into your home. The ratio of heat energy out to work energy input is referred to as a coefficient of performance, and in this instance would be 3J/1J or 3.
Heat pumps can use closed loop refrigerants like the example of your refrigerator, or in process applications, a process vapour can be compressed to a higher pressure and then condensed again to transfer heat. Doing so is called “mechanical vapour recompression” (MVR), and the process vapour- the vapour coming off the top of a distillation column for instance- becomes its own “refrigerant”, transferring heat by condensation in the reboiler. MVR schemes can have very high coefficients of performance indeed- sometimes in excess of ten. It all depends on the temperature difference, and also on the maximum temperature which must be low enough for both the working fluid and the equipment being used.
Conventional wisdom is that heat below about 200 C can be provided to industrial applications by heat pumping schemes with potentially excellent coefficients of performance. Fortunately, somewhere between 40 and 60% of industrial heat joules are used below 200 C. Above about 200 C, materials and compressor problems make the job harder, but development is ongoing and this limit is likely to soon be exceeded.
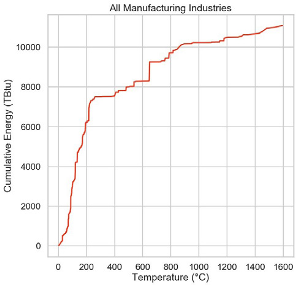
Above 200 C, the worst we can do is to basically convert electricity to heat with nearly 100% efficiency. We can make heat with resistance heaters to about 1000 C, and with microwaves, induction, plasma and electric arcs above that.
The notion that electric heating is difficult is more one of a lack of familiarity than one of technical difficulty. In a past where we used ¼ cent per kWh natural gas to make our electricity, it made no sense to use electricity to make heat except where convenience and cleanliness and control were king. Burning fuels was just cheaper in operating cost terms. And it was so much cheaper that it was worth a lot of bother coping with hot flue gas coming out of a high temperature furnace. Heat recovery steam generators, steam production etc. were a result of the need to not waste so much heat. In a future where we heat the things we want to heat directly with electricity, rather than indirectly using hot flue gas, most of this messy, maintenance intensive, capital-consuming heat recovery stuff simply goes away along with the toxic emissions of combustion flue gas.
Electric heating isn’t difficult. It just requires us to get the 800,000 year habit of “need heat- what can I burn?” out of our heads.
There are precious few applications which need fire rather than just heat, and those few that remain, can be satisfied with biofuels.
And no, there’s no imperative whatsoever to convert electricity into fuels like hydrogen in order to provide heat. That’s a sin against the 2nd law. We’ll need to be very rich and very desperate to do that. All e-fuels, including the simplest one (hydrogen), are exergy destroyers. And destroying exergy has is working in the wrong direction, just because we’re comfortable with it. That’s nonsense, and we must get over it as quickly as we can.
https://www.linkedin.com/pulse/breakthrough-electrolyzer-efficiency-paul-martin
Committest Thou Not the 2nd Sin of Thermodynamics!
Now that Father Paul has preached to you, thou shalt not confuse a joule of work with a joule of heat ever again. Go forth and sin no more against the 2nd Law! And remember- we need only replace useful energy services. All that heat wasted by your car’s radiator is going bye-bye for good. And for good, I mean for the good of the atmosphere and, ultimately, for the good of your wallet.
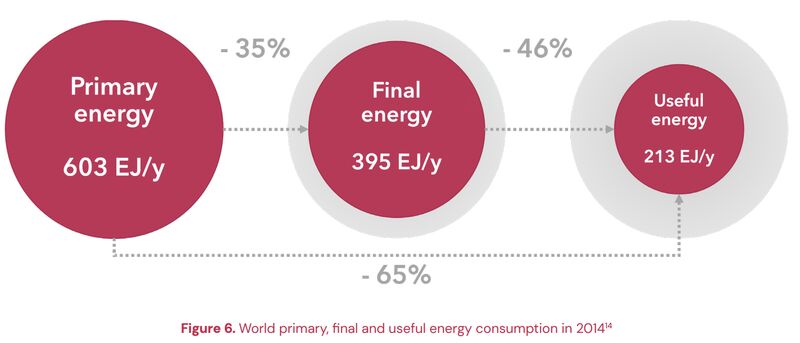
Disclaimer: a human wrote this, and humans are known to make mistakes from time to time. If you find an error, bring it to my attention with good references and I’ll fix it.
If however you simply don’t like what I’m saying because I’ve taken a dump on your pet idea, feel free to complain to Spitfire Research Inc., my employer, who will be happy to tell you to piss off and write your own article.